Advance Additive Manufacturing Technology
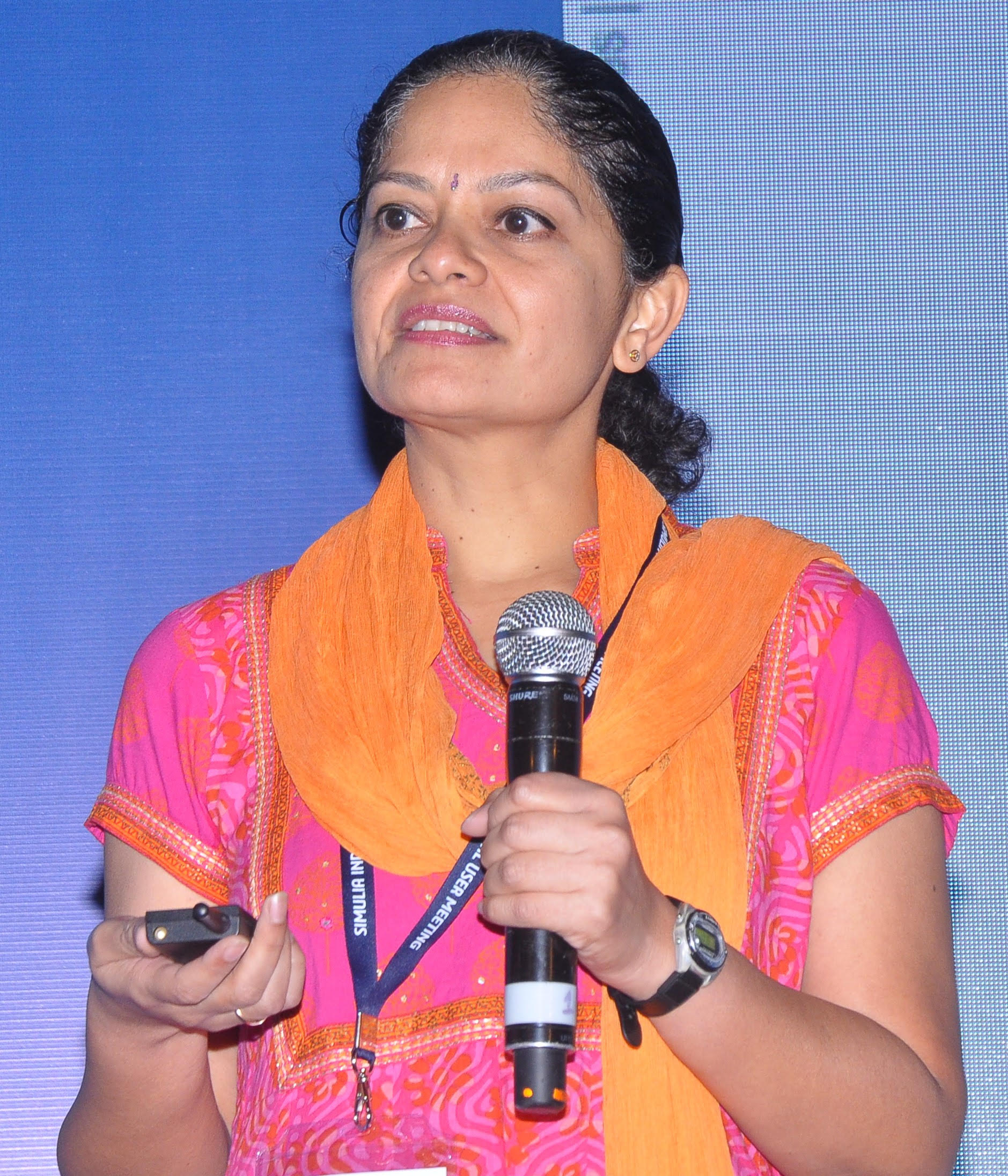
Simulation can help to provide a clear picture of the additive manufacturing process from the first to the last step
Additive manufacturing (AM) has gained popularity across the globe and has developed into a multi-billion-dollar industry that involves many materials and several process techniques. AM is maturing into a technology for the manufacture of metallic parts that are scientifically sound and commercially feasible.
Recent technology advances in additive manufacturing or 3D Printing as it is widely referred to, have even brought it to the desktop at home through the Maker movement. Additive manufacturing is making significant in-roads beyond rapid prototyping into industrial manufacturing of both tool and direct-part production. However, significant challenges still exist in the reliability and predictability of a number of AM processes that act as barriers to part certification and to much more widespread adoption in the industry.
Simulation can help to provide a clear picture of the additive manufacturing process from the first to the last step. While additive manufacturing offers more flexibility in terms of the design (CAD) and increased efficiency of the assembly compared to traditional fabrication methods, how can simulation help increase confidence in AM designs? That is, can we design parts such that they print right the first time? Can simulation help in reducing the number of components in an assembly? How to ensure the part strength is as expected?
There are a number of key areas where simulation can play an important role in 3D printing:
- Generating a functional design with highest strength-to-weight ratio
- Calibrating the material
- Optimizing the manufacturing process
- Validate the printed part for In-service performance
While conventional part manufacturing removes excess material through milling and machining, AM builds 3D complex parts via printing in precise layers along a software-guided path. In the best-case scenario, AM delivers improved product performance at a lower weight; it also enables more complex geometries and simplified fabrication or assembly, by offering designers freedom from traditional manufacturing constraints resulting in innovative designs.
With AM, parts can be created with a minimum of the material necessary to meet specified functional requirements. The creation of these kinds of designs is now feasible thanks to the proven technology of robust, nonlinear topology optimization that Finite Element Analysis (FEA) based simulation software such as SIMULIA Abaqus and SIMULIA Tosca offers.
A critical aspect of any AM process is to be able to characterize the underlying material that is being used. Typically, with metal alloys, for example, a high-intensity laser is applied to a powder bed, fusing the metal layer-by-layer to build the part. The metal melts locally and, as the heat source moves on, solidifies with the previous layer to create the fused part. The phase transformations, the cooling rates, and other machine-specific parameters such as print speed determine the metallurgy and the microstructures that are evolving in the heat-affected zones.
The optimized 3D printed parts can even be stronger than those made with traditional manufacturing methods such as casting, however, the variabilities in mechanical properties can be significant. Hence, there is a need to capture the multi-scale and multi-physics nature of this additive manufacturing process. Here is where the Abaqus user-subroutine framework is already enabling researchers and industry experts to model the physics of the micro-mechanics behavior during the processing stage while leveraging Abaqus as the global solver for the macro-behavior of the parts under different realistic loads
Aside from material characterization, the 3D-printing manufacturing process itself can introduce significant gaps between the as-designed and the as-manufactured part. In a typical simulation setup, the original design is without stresses or distortions and is assigned with standard material definitions.
However, during AM, which is generally a thermal-induced material phase transformation process, residual stress builds up, part distortions occur, and material property variations arise resulting in a need to define nonstandard material definitions. Further complications like build failures can occur, and Abaqus provides capabilities to monitor for build failure using mesh independent techniques like eXtended FEM (XFEM).
SIMULIA Insight is a powerful tool for design exploration and optimization of simulation processes. The tool has been used to study the effects of AM manufacturing process parameters including variability in material, temperature conditions, path speeds, etc. The tool can also be applied to reduce part distortions, and optimize process parameters to meet the in-service conditions of the part—whether those are static loads, dynamic loads, vibrations, that you are already solving using Abaqus.
Ultimately, the aim is to have durable components meeting the desired fatigue life under in-service loading conditions. Solutions like fe-safe can be used to estimate the fatigue life of a metal part. Its deep integration with Abaqus will enable fatigue life evaluation for additively manufactured components as the material data is developed in research.
So to answer our initial questions, yes, physics-based simulation has great potential to improve the quality and therefore support the growth of AM in every industry as the technology approaches maturity. As the VP of Technology Mr. Mike Corliss with Knust-Godwin, who makes high-tech equipment for Oil & Gas Industry, said – “Our customers are impressed with the quality of our additive parts. Working with our customers on their designs and using the 3DEXPERIENCE platform, we can show them the results that they’re looking for before we ever print a product.”
Author : Renuka Srinivasan, Director SIMULIA India – Dassault Systèmes